How To Calculate Formal Charge
To calculate the formal charge of an atom, we start by:
- evaluating the number of valence electrons (VE) the neutral atom has (e.g. 3 for boron, 4 for carbon, 5 for nitrogen, and so on). (note: this is also equivalent to the effective nuclear charge Zeff , the number of protons that an electron in the valence orbital “sees” due to screening by inner-shell electrons.)
- counting the number of non-bonded valence electrons (NBE) on the atom. Each lone pair counts as 2, and each unpaired electron counts as 1.
- counting the number of bonds (B) to the atom, or alternatively, counting the number of bonding electrons and dividing this by 2.
The formal charge FC is then calculated by subtracting NBE and B from VE.
FC = VE – (NBE + B)
which is equivalent to
FC = VE – NBE – B
The calculation is pretty straightforward if all the information is given to you. However, for brevity’s sake, there are many times when lone pairs and C-H bonds are not explicitly drawn out.
So part of the trick for you will be to calculate the formal charge in situations where you have to take account of implicit lone pairs and C-H bonds.
In the article below, we’ll address many of these situations. We’ll also warn you of the situations where the calculated formal charge of an atom is not necessarily a good clue as to its reactivity, which is extremely important going forward.
1. Formal Charge
Formal charge is a book-keeping formalism for assigning a charge to a specific atom.
To obtain the formal charge of an atom, we start by counting the number of valence electrons [Note 1] for the neutral atom, and then subtract from it the number of electrons that it “owns” (i.e. electrons in lone pairs, or singly-occupied orbitals) and half of the electrons that it shares (half the number of bonding electrons, which is equivalent to the number of bonds)
The simplest way to write the formula for formal charge (FC) is:
FC = VE – NBE – B
where
- VE corresponds to the number of electrons around the neutral atom (3 for boron, 4 for carbon, 5 for nitrogen, 6 for oxygen, 7 for fluorine)
- NBE corresponds to the number of non-bonded electrons around the atom (2 for a lone pair, 1 for a singly-occupied orbital, 0 for an empty orbital)
- B is the number of bonds around the atom (equivalent to half the number of bonding electrons)
It’s called “formal” charge because it assumes that all bonding electrons are shared equally. It doesn’t account for electronegativity differences (i.e. dipoles).
For that reason formal charge isn’t always a good guide to where the electrons actually are in a molecule and can be an unreliable guide to reactivity.
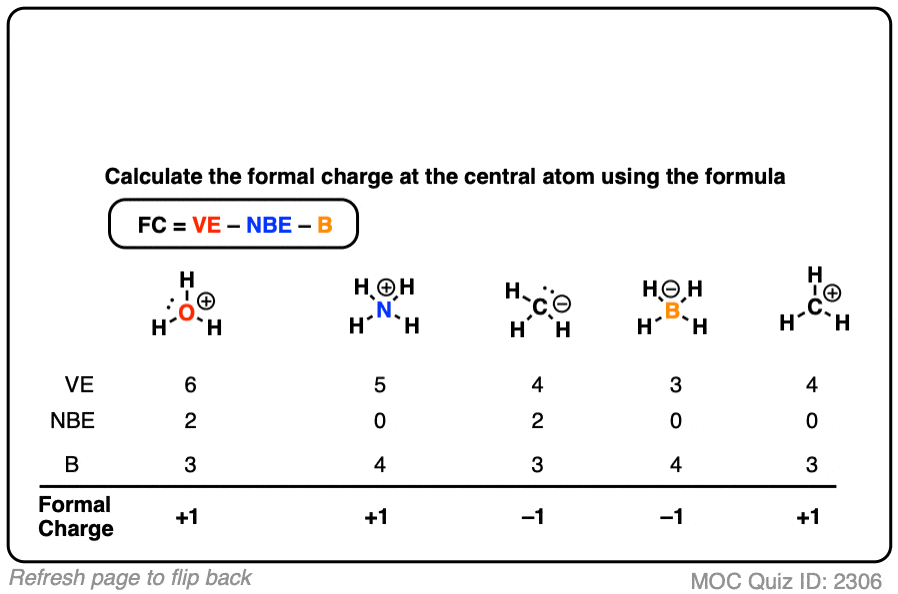
If that went well, you could try filling in the formal charges for all of the examples in this table.
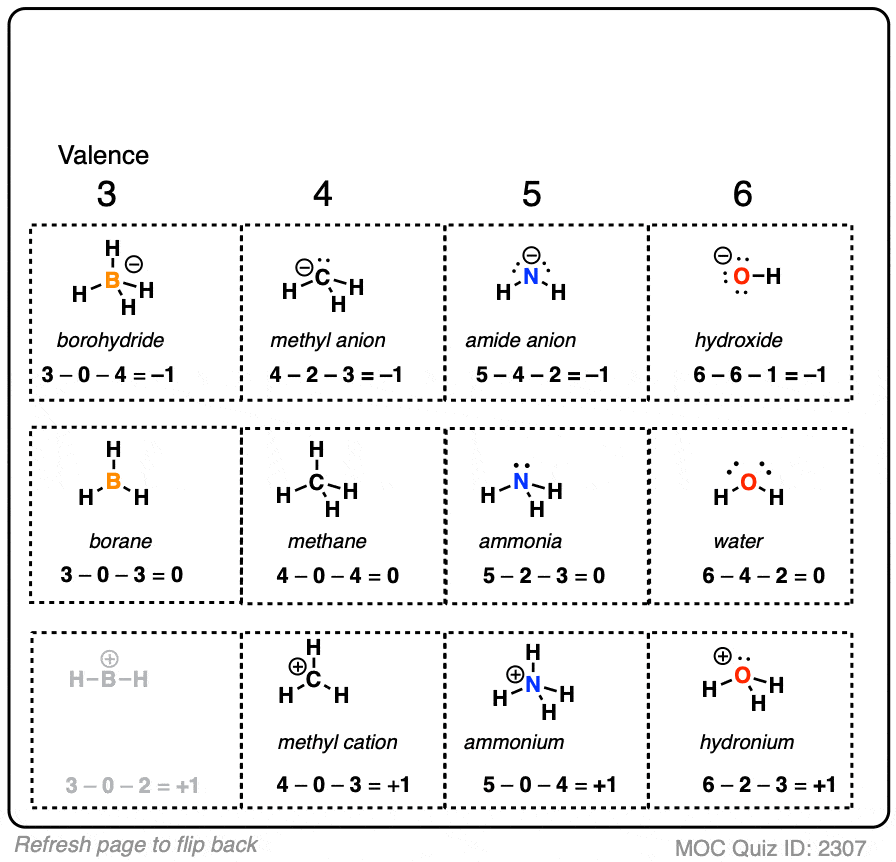
It will take some getting used to formal charge, but after a period of time it will be assumed that you understand how to calculate formal charge, and that you can recognize structures where atoms will have a formal charge.
Let’s deal with some slightly trickier cases.
3. Formal Charge Calculations When You Aren’t Given All The Details
When we draw a stick figure of a person and don’t draw in their fingers, it doesn’t mean we’re drawing someone who had a bad day working with a table saw. We just assume that you could fill in the fingers if you really needed to, but you’re skipping it just to save time.
Chemical line drawings are like stick figures. They omit a lot of detail but still assume you know that certain things are there.
- With carbon, we often omit drawing hydrogens. You’re still supposed to know that they are there, and add as many hydrogens as necessary to give a full octet (or sextet, if it’s a carbocation).
- If there is a lone pair or unpaired electron on a carbon, it’s always drawn in.
Oxygen and nitrogen (and the halogens) are dealt with slightly differently.
- Bonds to hydrogen are always drawn in.
- The lone pairs that are often omitted.
- Nitrogen and oxygen will always have full octets. Always. [Note 2 – OK, two exceptions]
So even when the lone pairs aren’t drawn in, assume that enough are present to make a full octet. And when bonds from these atoms to hydrogen are missing, that means exactly what it seems to be: there really isn’t any hydrogen!
Don’t get spooked out. Just count the electrons and the bonds, and that will lead you to the right answer.
4. Formal Charges and Curved Arrows
We use curved arrows to show the movement of electron pairs in reactions and in resonance structures. (See post: Curved Arrows For Reactions)
For example, here is a curved arrow that shows the reaction of the hydroxide ion HO(-) with a proton (H+).
The arrow shows movement of two electrons from oxygen to form a new O–H bond.
Curved arrows are also useful for keeping track of changes in formal charge. Note that the formal charge at the initial tail of the curved arrow (the oxygen) becomes more positive (from -1 to 0) and the formal charge at the final tail (the H+) becomes more negative (from +1 to 0).
When acid is added to water, we form the hydronium ion, H3O+.
But I’m willing to bet that at least a small percentage of you drew the arrow going to the positively charged oxygen.
What’s wrong with that?
There isn’t an empty orbital on oxygen that can accept the lone pair. If you follow the logic of curved arrows, that would result in a new O–O bond, and 10 electrons on the oxygen, breaking the octet rule.
Hold on a minute, you might say. “I thought oxygen was positively charged? If it doesn’t react on oxygen, where is it supposed to react?”
On the hydrogens! H3O+ is Brønsted acid, after all. Right?
Formal charge is ultimately a book-keeping formalism, a little bit like assigning the “win” to one of the 5 pitchers in a baseball game. [Note 3] It doesn’t take into account the fact that the electrons in the oxygen-hydrogen bond are unequally shared, with a substantial dipole.
So although we draw a “formal” charge on oxygen, the partial positive charges are all on hydrogen. Despite bearing a positive formal charge bears a partially negative electrostatic charge.
This is why bases such as HO(-) react at the H, not the oxygen.
Just to reiterate:
- Positive charges on oxygen and nitrogen do not represent an empty orbital. Assume that oxygen and nitrogen have full octets! [Note 2]
- In contrast, positive charges on carbon do represent empty orbitals.
6. Halogens
Positive formal charges on halogens fall into two main categories.
We’ll often be found drawing halonium ions Cl+ , Br+, and I+ as species with six valence electrons and an empty orbital (but never F+ – it’s a ravenous beast)
It’s OK to think of these species as bearing an empty orbital since they are large and relatively polarizable. They can distribute the positive charge over their relatively large volume.
These species can accept a lone pair of electrons from a Lewis base, resulting in a full octet.
Cl, Br, and I can also bear positive formal charges as a result of being bonded to two atoms.
It’s important to realize in these cases that the halogen bears a full octet and not an empty orbital. They will therefore not directly accept a pair of electrons from Lewis bases; it’s often the case that the atom adjacent to the halogen accepts the electrons.
7. Conclusion
If you have reached the end and did all the quizzes, you should be well prepared for all the examples of formal charge you see in the rest of the course.
- Formal charge can be calculated using the formula FC = VE – NBE – B
- Line drawings often omit lone pairs and C-H bonds. Be alert for these situations when calculating formal charges.
- Positively charged carbon has an empty orbital, but assume that positively charged nitrogen and oxygen have full octets.
- The example of the hydronium ion H3O+ shows the perils of relying on formal charge to understand reactivity. Pay close attention to the differences in electronegativity between atoms and draw out the dipoles to get a true sense of their reactivity.